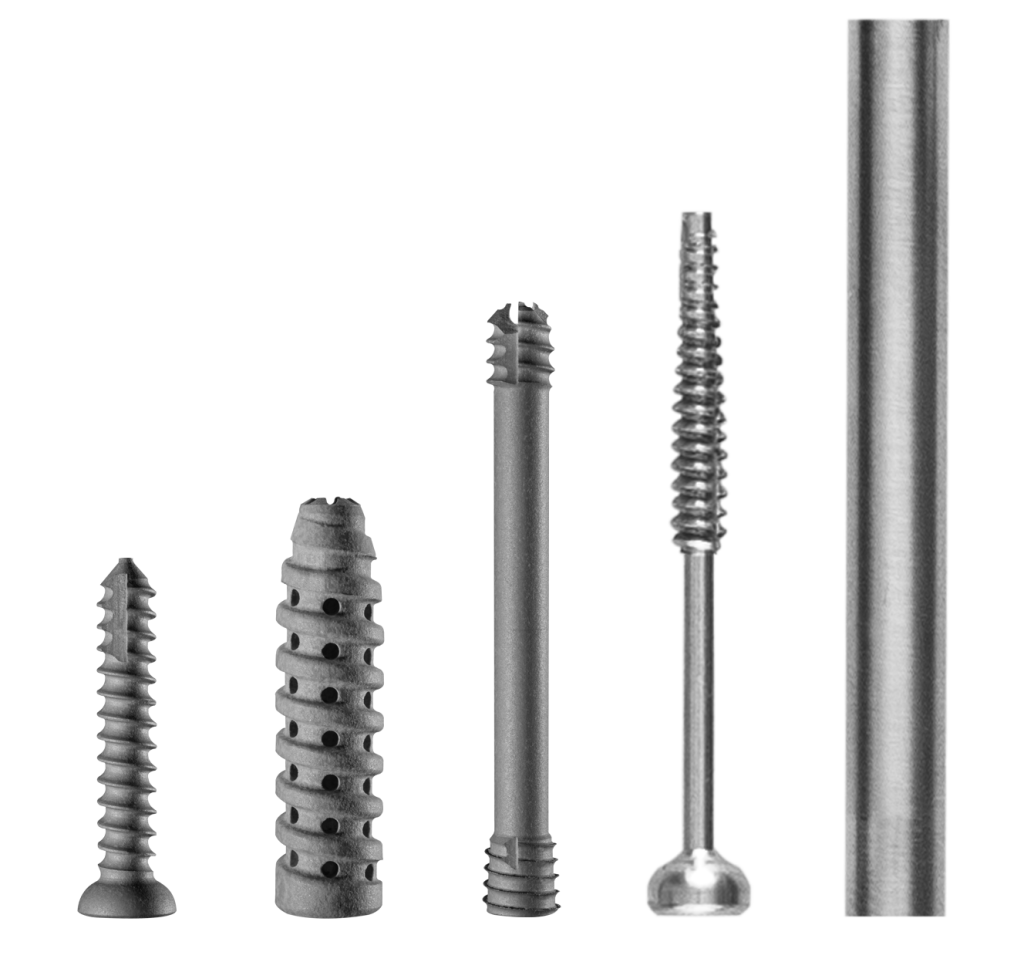
Overview
The use of magnesium alloys as biodegradable implant materials holds immense promise, driven by their biocompatibility, impressive mechanical properties, and potential for degradation in vivo. However, their rapid deterioration poses a significant challenge, limiting their practical applications. This project aims to address this challenge by thoroughly investigating the processing-structure-property relationships of magnesium alloys, particularly focusing on their mechanical integrity and degradation behavior.
Objectives
- Optimization of Processing Conditions: Through systematic experimentation involving over thirty processing conditions, identifying the optimal combination of processing parameters that achieve high-strength properties while minimizing corrosion rates in magnesium alloys. This work is carried out in collaboration with Mr. Adam Griebel from Fort Wayne Metals.
- Characterization of Microstructural Changes: Employing advanced characterization techniques such as X-ray diffraction (XRD), microscopy (optical and scanning electron microscopy – SEM, and Transmission Electron Microscopy) assess microstructural changes in magnesium alloys post-thermal and thermomechanical processing.
- Understanding Mechanical and Degradation Properties: Investigating the mechanical (hardness, quasi-static tension, and fatigue) and corrosion properties in Magnesium alloys post-thermal and thermomechanical processing.
- Identifying Influential Microstructural Factors: Identifying key microstructural factors such as grain boundary characteristics, strain hardening effects, texture, dislocation density, and precipitates that influence the mechanical properties and degradation behavior of magnesium alloys.
- Machine Learning Analysis: We employ advanced statistical methods including Pearson correlation coefficient analysis and feature selection machine learning techniques such as XGBoost, LASSO, and Bayesian Optimization, in collaboration with Clancy Group to unravel the intricate relationships between microstructural properties and the mechanical and degradation properties. Using their input, develop ideal processing conditions leading to an optimal combination of mechanical and degradation properties.
- In-vivo studies: In collaboration with Prof. Roger Guillory, we conduct a wide range of in-vivo implantations to understand the degradation and inflammation behavior.
- Computer-aided design and finite element modeling of implants: Design magnesium-based screws and plates and model their mechanical and degradation behavior using finite element analysis in collaboration with Prof. Jamie Guest and Dr. Amiethab Aiyer.
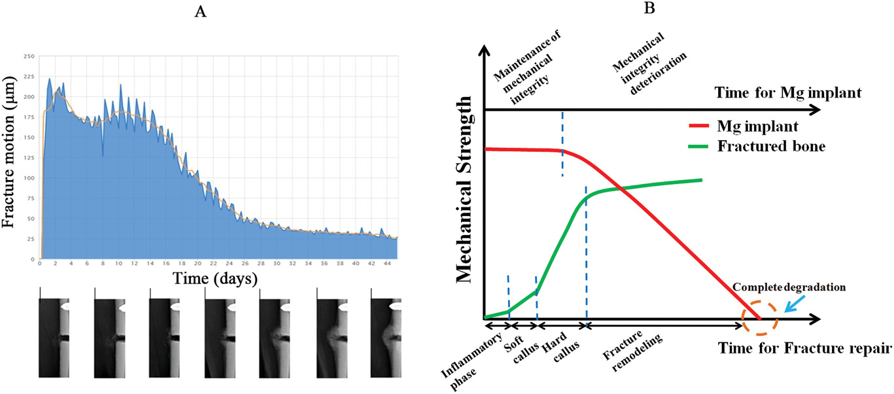
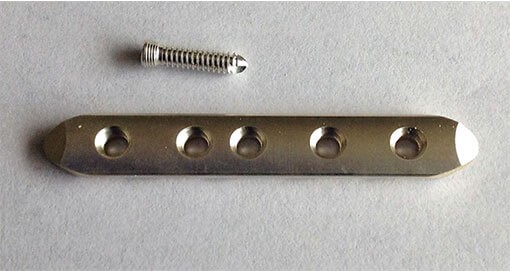
Expected Outcomes
- Insights into Processing-Structure-Property Relationships: This project will provide valuable insights into the complex relationships between processing parameters, microstructural characteristics, and mechanical properties of magnesium alloys, particularly regarding their biodegradability and biocompatibility.
- Optimized Alloy Design: By identifying the most influential factors and optimal processing conditions, this research will contribute to the development of superior biodegradable implant materials with improved mechanical integrity and prolonged service life in vivo.
- Data-driven Approach: The application of advanced statistical and machine learning techniques will enable a data-driven approach to understanding and optimizing the properties of magnesium alloys, facilitating the design of next-generation biocompatible implant materials.
Significance
The successful development of biodegradable implant materials based on magnesium alloys has the potential to revolutionize the field of orthopedic implants, offering patients improved biocompatibility, reducing the risk of long-term complications, and ultimately enhancing their quality of life. Furthermore, the insights gained from this research could have broader implications for designing and engineering biodegradable materials in various biomedical applications.